Cover Story
Point to Point
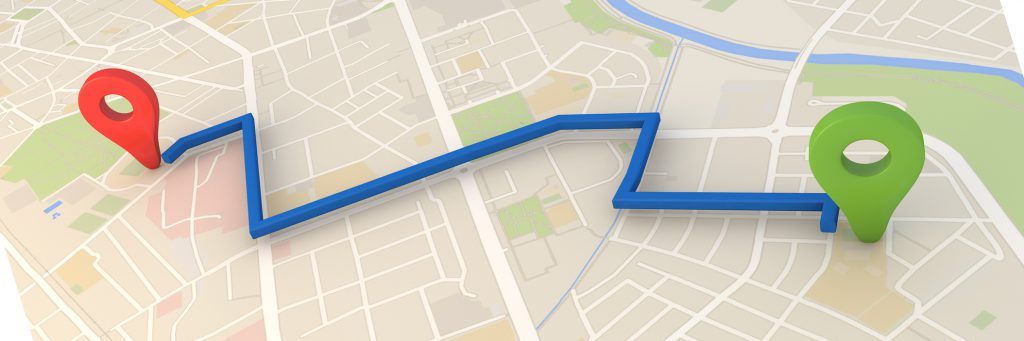
Twenty years ago, the US Air Force Space Command declared the Global Positioning System fully operational, and within a few years it would become to civilians around the world what the compass was to early mariners. Today, this satellite technology is tucked in smartphones and dashboard-mounted devices, with a friendly electronic voice guiding us anywhere we need to go. Yet this technological convenience hasn’t stopped psychological scientists from exploring the sophisticated navigational skills that emerge from our own biology.
Over the past few decades, psychological research has revealed a complex mapping system within the brain, and new aspects of this system have been discovered within just the last few years. This research has become a highly-lauded endeavor for the field: The 2014 Nobel Prize in Physiology or Medicine went to three European psychological scientists, John O’Keefe (University College London) and wife-and-husband team May-Britt Moser and Edvard Moser (Norwegian University of Science and Technology), for their groundbreaking studies on the brain’s own “GPS system.”
In experiments with both animals and humans, psychological scientists have generated an understanding of the brain’s own GPS capabilities, from the level of individual cells all the way up to higher level cognitive networks. And this study of spatial orientation, with its focus on the mind’s ability to encode routes for future reference, may spawn new insights into episodic memory and the cognitive disorders that compromise it.
Brain Mapping Links
Observer sidebar on “Charging Up the Inner GPS”
Recording of activation of hippocampal place cells in a rat (1:14)
Recording of activation of hippocampal place cells in a rat (0:30)
2014 Nobel Prize in Physiology or Medicine lecture (2:49:27)
Yellow Cab session demonstration (downloadable .mov file; 3:41)
Virtual Tübingen Web page
Hugo Spiers’ art and architecture
A Trio of Brain Cells
Much of the foundational work on navigational processing involved animal research, which, over time, unveiled a pattern of cellular activity that controls spatial orientation and direction. O’Keefe’s work dates back to the 1970s, when, using microelectrodes attached to the skulls of rats, he identified hippocampal nerve cells that were always active when the rats were in a specific location. These “place cells” seemed to register not only what the rats saw, but also what they did not see, giving them a complete picture of their surrounding environment. O’Keefe and his colleague APS Fellow Lynn Nadel (University of Arizona) outlined their theory of this mapping system in their influential 1978 book, The Hippocampus as a Cognitive Map.
Other psychological researchers have built on this work to construct a detailed picture of the brain’s mapping system. In 1990, psychological scientists Jeffrey Taube (Dartmouth College), the late Robert Muller, and James Ranck (SUNY Downstate Medical Center), then in the department of physiology at the State University of New York Health Sciences Center, published a study of another type of brain cell that contributes to our sense of spatial orientation — the “head direction cell.” These head direction cells, they reported, fire when an animal’s head points in a specific direction, with different neurons firing for different head orientations.
And in 2005, May-Britt Moser and Edvard Moser, who had worked with O’Keefe as visiting postdoctoral fellows in his laboratory, pinpointed another important component of the brain’s navigation system when they identified a third type of nerve cell, the “grid cell,” which assists rats with positioning and remembering distances traveled. As rats moved about a laboratory, these cells — located in the entorhinal cortex, a region of the brain essential for navigation and memory — lit up in unique hexagonal patterns depending on the rats’ locations.
These results, paired with O’Keefe’s earlier discoveries, showed how place, head direction, and grid cells make it possible for rats to determine their spatial orientation and to navigate their environment.
Science also has generated evidence that these navigational abilities are innate. Studies led by behavioral neuroscientist Rosamund Langston, now at the University of Dundee, Scotland, along with Thomas Wills and other colleagues at University College London, found that head direction, place, and grid cells appear to function in rodents very early in life.
Langston and colleagues recorded brain activity in rat pups as they began exploring their environment. They found that the three types of navigational neurons were operating from the start and that they matured in a specific order: Head direction cells matured first, followed by place cells, and then grid cells. All three navigational cell types were fully matured by the time the pups were a month old.
These findings suggested that the rat brain has a foundation for a sense of space and direction that grows with exploratory stimulation.
‘Remapping’
More recently, scientists like Taube have explored the signaling of these cells in new ways. Taube’s lab has recorded the activity of cells in a rat’s brain that appears to guide the animal from one place to another. In a study published in 2012 in Nature Neuroscience, he and postdoctoral researcher Stephane Valerio (now at Université Bordeaux Segalen in France) described the responses of the rat’s spatial navigation system when it makes an error and arrives at an unintended destination.
According to results of their experiments, when a rat just slightly misses the target endpoint, the cells will reset to fix on landmarks the animal can identify in its landscape. But if the rat becomes disoriented and makes a large error in its quest for home, the animal will create a whole new cognitive map with a permanent shift in the directional firing pattern of the head direction cells. In other words, the rat’s brain “remaps.”
Taube acknowledges that others have talked about remapping and resetting, treating these functions as if they were the same process. “What we are trying to argue in this paper is that they are really two different, separate brain processes, and we demonstrated it empirically,” he says. “To continue to study spatial navigation, in particular how you correct for errors, you have to distinguish between these two qualitatively different responses.”
Sense of Direction
A number of studies indicate that other mammals, including humans, have a spatial-coding system in the brain similar to what rats possess. Psychological scientist Arne Ekstrom first reported on human place cells in 2003 as part of his dissertation at Brandeis University. Ekstrom, an associate professor of psychology at University of California, Davis, published his findings in Nature. And in a 2013 article published in Nature Neuroscience, a team led by Joshua Jacobs, who runs Columbia University’s Cognitive Brain Dynamics Lab, reported on the identification of human grid cells.
Experiments with human subjects have further elucidated the concept of navigation, including the role of “path cells,” which indicate directional motion in travel. Jacobs and his colleagues published a 2010 study on path cells in the Proceedings of the National Academy of Sciences. That multidisciplinary research, which Jacobs conducted as part of his dissertation work at the University of Pennsylvania, demonstrated that during navigation in a virtual environment, an individual path cell activates when a person is driving clockwise around a circular road and is silent when the person is moving counterclockwise. By representing direction, these path cells complement the place cells that encode location.
Under the direction of APS Fellow Michael Kahana, Jacobs and his colleagues had neurosurgical patients play a virtual-navigation video game called Yellow Cab. Using a handheld joystick, patients “drove” a taxi through a circular virtual town, either clockwise or counterclockwise, to a randomly selected destination. With the use of electrodes implanted in the patients’ brains, researchers were able to record the firing of more than 1,400 neurons and examine the relationship between brain activity and behavior in each patient.
This research showed that path cells work in tandem with place cells to tell us where we are and where we’re headed.
Lessons in London
Can individual navigational ability be sharpened with experience, or is it largely fixed? Some of the answers can be found in the brains of London taxi drivers. More than a decade ago, researchers discovered that these cabbies, who have to navigate one of the most labyrinthine street systems in the world, have atypical brain characteristics. Specifically, their posterior
hippocampi — a brain region associated with spatial memory — are larger than those of other people.
Psychological scientist Hugo Spiers, University College London, and colleagues recently mimicked the London cabbies’ experience in a virtual experiment and concluded that the brain processes underlying navigational ability are more complex than previously recognized. The study showed that we calculate two types of distance in separate areas of the brain.
Spiers and his team recruited volunteers and asked them to memorize maps of London’s Soho district, famous for its winding streets and complex junctions. The participants then took a guided tour through that neighborhood. The following day, Spiers and his team put the volunteers in an fMRI scanner and had them view various videos of a trip through the busy Soho streets. In some of the videos, a GPS guided the participants through the district to specified landmarks. But in others, participants were tasked with navigating on their own to reach the destination. The researchers analyzed their brain activity during different stages of the journey: setting course for the destination, keeping track of the destination while traveling, and decision-making at street junctions.
The team found that when the volunteers first had to think about their destination, the straight-line distance to that target location was signaled by activity in the entorhinal cortex. But during the rest of the journey, as they kept track of their direction and made decisions at street corners, activity in the posterior hippocampus, also centrally involved in navigation and memory, kept track of the distance along the route needed to reach the destination.
This, to Spiers and his colleagues, provided some explanation for the distinct brain characteristics of the London cabbies.
“Our results suggest that it is the daily demand on processing paths in their posterior hippocampus that leads to the impressive expansion in their gray matter,” Spiers said.
The results of Spiers’s study also reveal what happens in our brain when we use a GPS device or application to get to a destination. The fMRI measures showed relatively decreased activity in the entorhinal cortex and posterior hippocampus when participants used GPS-like instructions to reach their endpoints and also a general decrease in overall brain activity.
The Downside of GPS
In fact, some scientists have raised concerns that increasing dependence on new navigational technologies may lead our innate mapping skills to atrophy. For example, researchers like psychologist Stefan Münzer, University of Mannheim in Germany, and cognitive scientist Toru Ishikawa, University of Tokyo, have found in experiments that although people using GPS devices — which constantly reorient themselves based on the user’s location — make fewer navigational mistakes en route to their destinations than people who use traditional maps, they don’t remember as much about the landmarks they passed on the way. In other words, they aren’t encoding the very markers that could be used in subsequent trips to the destination. Think about the number of times you’ve had to use a GPS to guide you to a place you’ve visited more than once.
A study published in Psychological Science shed more light on the potential cognitive effects of technological navigation. Authors Julia Frankenstein, Betty J. Mohler, Heinrich H. Bülthoff, and Tobias Meilinger, who collaborated at the Max Planck Institute for Biological Cybernetics in Tübingen, Germany, found that in a non-GPS world, people often rely on their memory of maps for spatial reference.
In the study, 26 people who had lived in Tübingen for at least 2 years donned virtual-reality headsets that displayed a three-dimensional photorealistic model of the town. The participants started at locations that were familiar to them, but were surrounded by a fog that masked all but their nearest surroundings. They then had to point to an invisible location — say, the main gate of the university or the fire station. The scenes changed, and so did the participant’s viewing direction (e.g., facing north, facing east). Participants pointed most accurately when facing north and got worse the further they deviated from north.
The only explanation the researchers could offer was that the participants had all seen, and internalized, a map of Tübingen at some point, and Western maps are all oriented the same way: north on top. This finding suggests that, for certain tasks like pointing to a location, we rely on a mental map as a quick way to solve spatial puzzles.
But in actual on-the-ground travel, people appear to rely on different reference points. In a follow-up experiment conducted a week later — again using a virtual 3-D model of the city — the same participants “walked” along a familiar route to a distant target, indicating their route decisions on a keyboard. Their response times and errors in recalling the route had no correlation with those that resulted from the earlier experiment that used pointing, the researchers reported. Further, participants made fewer routing errors when asked to respond from a ground-level, navigation-based perspective rather than a constant aerial perspective.
“The implication is that, within their everyday environment, people use map- or navigation-based knowledge according to which best suits the task,” the research team said.
These scientists warn that increasing reliance on GPS devices could eventually erase our memorized maps. They emphasize that we need to continuously practice our spatial abilities to keep them sharp, even if we have tools that do the work for us.
“If somebody doesn’t care to learn the environment, that’s totally fine,” Meilinger said. “But they shouldn’t complain if their mobile is not working and they are completely lost.”
In the future, this ever-blossoming understanding of the brain’s navigational capacity could advance the understanding and perhaps treatment of symptoms that often accompany Alzheimer’s disease and other forms of dementia. Brain imaging studies have shown that the hippocampus and entorhinal cortex are among the first regions to show compromise in people diagnosed with Alzheimer’s disease. The navigation studies provide some explanation as to why patients with certain forms of dementia struggle to find their way and become lost — even in familiar environments.
Edvard Moser will be speaking as part of the Presidential Symposium at the 2016 APS Annual Convention, May 26–29 in Chicago.
References and Further Reading
Ekstrom, A. D., Kahana, M. J., Caplan, J. B., Fields, T. A., Isham, E. A., Newman, E. L., & Fried, I. (2003). Cellular networks underlying human spatial navigation. Nature, 425, 184–188. doi:10.1038/nature01964
Frankenstein, J., Mohler, B. J., Bülthoff, H. H., & Meilinger, T. (2012). Is the map in our head oriented north? Psychological Science, 23, 120–125. doi: 10.1177/0956797611429467
Hafting, T., Fyhn, M., Molden, S., Moser, M.-B., Moser, E. I. (2005).Microstructure of a spatial map in the entorhinal cortex. Nature, 436, 801–806. doi:10.1038/nature03721
Howard, L. R., Javadi, A. H., Yu, Y., Mill, R. D., Morrison, L. C., Knight, R., … Spiers, H. J. (2014). The hippocampus and entorhinal cortex encode the path and euclidean distances to goals during navigation. Current Biology, 24, 1331–1340. doi: 10.1016/j.cub.2014.05.001
Ishikawa, T., Fujiwara, H., Imai, O., & Okabe, A. (2008). Wayfinding with a GPS-based mobile navigation system: A comparison with maps and direct experience. Journal of Environmental Psychology, 28, 74–82. doi:10.1016/j.jenvp.2007.09.002
Jacobs, J., Kahana, M. J., Ekstrom, A. D., Mollison, M. V., & Fried, I. (2010). A sense of direction in human entorhinal cortex. Proceedings of the National Academy of the Sciences of the United States of America, 107, 6487–6492. doi: 10.1073/pnas.0911213107
Jacobs, J., Weidemann, C. T., Miller, J. F., Solway, A., Burke, J. F., Wei, X.-X. … Kahana, M. J. (2013). Direct recordings of grid-like neuronal activity in human spatial navigation. Nature Neuroscience, 16, 1188–1190. doi:10.1038/nn.3466
Meilinger, T., Frankenstein, J., & Bülthoff, H. H. (2013). Learning to navigate: Experience versus maps. Cognition, 129, 24–30. doi: 10.1016/j.cognition.2013.05.013
Münzer, S., Zimmer, H. D., Schwalm, M., Baus, J., & Aslan, I. (2006). Computer-assisted navigation and the acquisition of route and survey knowledge. Journal of Environmental Psychology, 26, 300–308. doi: 10.1016/j.jenvp.2006.08.001
O’Keefe, J., & Nadel, L. (1978). The hippocampus as a cognitive map. Oxford, United Kingdom: Oxford University Press.
Valerio, S., & Taube, J. S. (2012). Path integration: How the head direction signal maintains and corrects spatial orientation. Nature Neuroscience, 15, 1445–1453. doi:10.1038/nn.3215
APS regularly opens certain online articles for discussion on our website. Effective February 2021, you must be a logged-in APS member to post comments. By posting a comment, you agree to our Community Guidelines and the display of your profile information, including your name and affiliation. Any opinions, findings, conclusions, or recommendations present in article comments are those of the writers and do not necessarily reflect the views of APS or the article’s author. For more information, please see our Community Guidelines.
Please login with your APS account to comment.